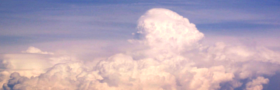
Fig. 1: Convective cloud with developing ice phase.
Fig. 1: Convective cloud with developing ice phase.
Under atmospheric conditions, cloud droplets form when the air is saturated or supersaturated with water. The excess water does not form pure water droplets, but condenses on so-called cloud condensation nuclei (CCN). Whether a particle can serve as a CCN under the given atmospheric conditions depends on both the particle properties (especially size and chemical composition) and the atmospheric dynamics (mainly the vertical velocity). Almost all airborne particles above a certain size (50-80 nm) are potential CCN. In general, the number of droplets in a cloud increases when
the CCN number increases
the particles become larger
the particles are water soluble
the updraft velocity increases
Changes in cloud droplet formation influence cloud properties such as solar radiation scattering and precipitation formation by coalescence. Microphysical models (e.g. SPECS) can be used to investigate the extent to which a cloud reacts to changes in the aerosol population (e.g. in terms of droplet numbers, liquid water mass, precipitation, etc.).
The detailed description of cloud microphysics is realized with a spectral approach (Simmel et al., 2002; Simmel and Wurzler, 2006). This means that the number size distributions of hydrometeors (aerosol particles, droplets, ice particles) are discretized in high resolution with respect to their mass (e.g. 66 size classes for the range from 1 nm to several mm). In this way, the relevant microphysical processes of the liquid (drop nucleation, condensation, coalescence, drop bursting) and solid (ice nucleation, freezing processes, riming) phases can be described explicitly, i.e. there is no need to rely on parameterizations.
A particular focus is on the description of ice nucleation. Supercooled water droplets would typically freeze in the atmosphere only at temperatures from -35 °C to -40 °C and lower by homogeneous nucleation. However, ice particles are observed at much warmer conditions (sometimes only a few degrees below 0 °C, often from -15 °C and colder), formed by heterogeneous ice nucleation processes, i.e. involving mostly insoluble particles called 'ice nucleating particles' (INP). In the atmosphere, four of these processes are relevant: Deposition, condensation, immersion and contact freezing. Among these, the immersion freezing and contact freezing processes are typically the most efficient. In immersion freezing, freezing is initiated by an INP located inside a supercooled droplet, whereas in contact freezing, a supercooled droplet collides with a suitable INP, thus initiating freezing from the outside. The freezing temperatures and efficiencies are highly dependent on the type of INP involved. For example, biological particles are significantly more effective than mineral particles or black carbon, particularly in immersion freezing. Contact freezing typically starts at even warmer temperatures, and the differences between biological and mineral INPs are smaller (Diehl and Wurzler, 2005; Diehl et al., 2006).
Sensitivity studies as well as realistic simulations are carried out. The cloud microphysics can be described within the framework of an air parcel model (also due to the high computational time requirement due to the many variables and elaborate process descriptions). However, spectral microphysics has also been implemented in a cylindrically symmetric Asai-Kasahara model (Simmel et al., 2015) as well as in the COSMO model of the German Weather Service (DWD) (Grützun et al., 2008).
The current efforts in microphysics model development are directed towards increasing the degree of realism of the model simulations in order to enable comparison with measurements using modern remote sensing methods. Realistic models have a high degree of complexity and allow multiple interactions between aerosol, cloud droplets and cloud ice, which influence the formation of precipitation. For both CCN and INP, the importance of the aerosol influence on cloud microphysics can be compared with other driving forces such as the underlying general dynamics, turbulence or other influences.
Fig. 2: Dependence of cloud droplet number on the number of cloud condensation nuclei (CCN) and vertical velocity for a given CCN size distribution and chemical composition.
Fig. 3: Time evolution of the spectrum of the combined droplet and ice crystal mass mixing ratio for a model run for the CyCare campaign. While the cloud droplets and ice crystals (1-100 µm) are located at about 3-7 km (middle part of the spectrum), large droplets and ice crystals (> 100 µm) can be seen at the right edge of the spectrum, which have sufficient falling velocities to reach the ground as precipitation.