- i) Cooperation with PSI glaciologists in Switzerland, to measure INP in samples of two Arctic ice cores; no significant increase in INP concentrations compared to today was found in the period studied (past 500 years up t0 1989); on short time scales (samples than were less than one year long), INP concentrations showed a significantly higher scatter compared to perennial samples; oberall, the observed scatter in the data remained within that observed in current data (Hartmann et al., 2019).
For the primary formation of ice crystals in clouds in the temperature range between 0°C and roughly -38°C, catalysts are needed which can initiate the nucleation of ice in a liquid, supercooled drop. Atmospheric particles that can act as such catalysts are called ice nucleating particles (INP).
Atmospheric concentrations of INP have been determined by TROPOS in a series of measurement campaigns at various locations around the world. Atmospheric filter samples were collected and evaluated in the laboratory. Off-line instrumentations were used, which can provide data in the temperature range from 0°C to approx. -25°C (the statements below therefore generally refer to this temperature range). The locations at which examinations were done (in the sequence listed below) include the Arctic, Antarctica, gobal oceans, southern Chile, Cape Verde/Cabo Verde, Beijing, Maumee River und Lake Erik (USA), Cyprus.
The obtained data are among others important input parameters for climate models and can help to improve future model calculations.
The following investigations and observations, shown separately for different regions of the Earth, were made:
Arctic
- ii) Cooperation with partners from four different Arctic measuring stations (Alert, Ny Alesund, Utqiagvig (formerly Barrow), Villum Research Station); yearly variations of INP concentrations were found, with high values in summer and lower values in winter, which was particularly pronounced for very ice-active INP. Possible sources for the highly ice-active INP (presumably biogenic in origin) were identified in the Arctic (both terrestrial and maritime sources) (Wex et al., 2019).
- iii) Sample collection at Villum Research Station, both ground-based and on board the Polar 5 aircraft of the Alfred-Wegener-Institute in the framework of the PamArcMip campaign in March/April 2018. For days when flights were made at low altitudes above the mostly (but not completely) frozen ocean, elevated INP concentrations were found, while all other samples collected at higher altitudes showed low INP concentrations. For elevated INP concentrations, a biogenic origin from open leads and polynyas is assumed (Hartmann et al., 2020).
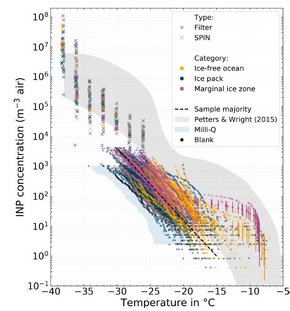
Figure from Hartmann et al. (2021), showing INP concentrations as obtained during the Polarstern cruise.
- iv) Sample collection during a voyage of the German research vessel Polarstern within the framework of (AC)3 (project B-04) in May - July 2017 - the observed atmospheric INP concentrations vary over 2 to 3 orders of magnitude at any particular temperature, are for the most part lower than at mid-latitudes, and cannot be explained by marine sources without enrichment factors of at least 104. But source attribution was not clearly possible and local marine sources must be considered. Initial data were published as part of a campaign review ((Wendisch et al., 2019), while Hartmann et al. (2021) discusses the results in detail.
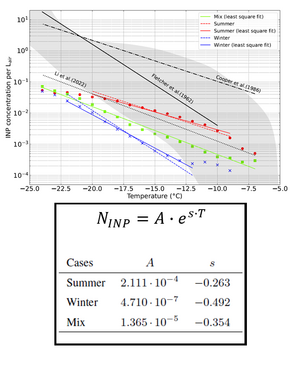
Parameterizations for three typical INP spectra given in Sze et al. (2022), in comparison to literature data.
- v) In North Greenland, at Villum Research Station, we were able to establish a two-year long series of INP data from filter samples with the help of our Danish partners. The data clearly show that the observed high INP concentrations in summer can be attributed to biogenic INP (proteins). Parameterizations for three typical INP spectra could be derived (Sze et al., 2022):
- for the months with high INP concentrations (June - October, "summer"), occurrence approx. 60% of the time in these months
- for the months with low INP concentrations (December - March, "winter"), occurrence about 60% of the time in these months
- for intermediate cases, occurrence all year round about 40% of the time (April and May and November are transition months)
Antarctica
- i) Examinations were made for the Southern Ocean region, based on data collected during an Antarctic circumnavigation during the ACE-SPACE campaign from November 2016 to March 2017 (for campaign overview see Schmale et al., 2019).
INP concentrations were generally low. They were particularly low in air masses that had only been over the ocean or over Antarctica during the period studied. Air masses that had crossed other land masses (except Antarctica) showed significantly higher INP concentrations (Tatzelt et al., 2022).
- ii) Some first data for samples from the long-term filter collection at AWI's Antarctic Neumayer Measuring Station show INP concentrations similar to those described for the Antarctic Ocean in literature. One of the samples also contained INP that can be attributed to biogenic proteins, as they could be destroyed by heat.
Global oceans
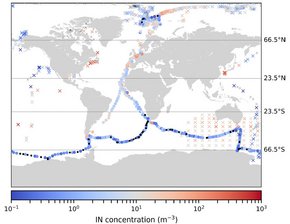
Bild aus Welti et al. (2020) mit atmosphärischen INP Konzentrationen während verschiedener Schiffsmesskampagnen.
- INP concentrations from ship measurement campaigns (both our own and from literature) are 1 to 2 orders of magnitude lower than those from measurements performed on continents. No contribution to INP from ship emissions in the temperature range above -36°C was observed (Welti et al., 2020).
Southern Chile
- As part of the DACAPO-PESO project, long-term in-situ aerosol data and filters are being collected to determine INP concentrations in southern Chile (Cerro Mirador west of Punta Areanas at an altitude of about 600m). No annual variation is observed, but INP concentrations are similar throughout the year as e.g. on Cape Verde (Cabo Verde). These INPs are mostly of biogenic origin (proteins). The INPs appear to be of continental origin, and precipitation seems to be the most important driver for enhancing terrestrial emissions of INPs (Gong et al., 2022).
Cape Verde islands / Cabo Verde
- 4 years of continuous filter samples (2009-2013) showed increased INP concentrations in combination with Sahara dust for INP active at lower temperatures (< -16°C). A source assignment for INP active at higher temperatures was not possible. A log-normal distribution of the INP concentrations, as observed at different separate temperatures, suggests that the INP do not originate from local sources, but from a number of different sources whose contributions were randomly turbulently mixed during transport (Welti et al., 2018).
- Within the framework of MarParCloud, INP concentrations were determined for various samples: Ocean water, oceanic surface micro layer (SML), in cloud water and in the air at two measuring stations (sea level and at an altitude of 700m on a mountain). Atmospheric INP are mostly supermicron in size, highly ice-active INP are of biogenic origin, and the observed atmospheric INP concentrations cannot be explained by marine sources unless there would be enrichment factors of at least 104 (Gong et al., 2020).
Beijing
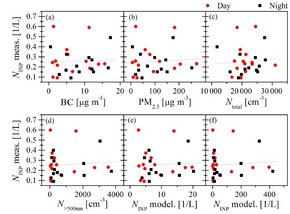
Figure from Chen et al. (2018) showing INP concentrations measured in Beijing in comparison to other parameters.
- Investigation of INP in atmospheric aerosol that was, at times, strongly influenced by anthropogenic pollution; it was found that no additional INP were introduced into the atmosphere even during phases of strong air pollution, i.e. that anthropogenic particles in Beijing did not function as INP in the atmosphere in the investigated temperature range (Chen et al., 2018).
Maumee River and Lake Erie (US)
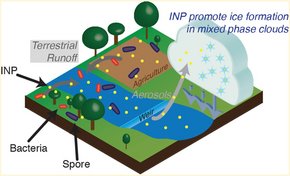
Conceptualized sketch from Knackstedt et al. (2018), showing the circulation of biogenic INP in nature.
- The farmland surrounding Maumee River was identified as the source of biogenic INP in the river (possibly the soil fungus Mortierella alpina). In Lake Erie (into which the Maumee River flows) additional different biogenic INP were found. Atmospheric INP concentrations above a weir were elevated compared to the surrounding area, suggesting an input of INP from the river (Knackstedt et al, 2018).
Cyprus
- Filter samples were collected both in the framework of a joint INUIT/BACCHUS-campaign (April 2016) as well as during A-LIFE (April 2017). Measurements of different devices for determining the INP concentration were consistent in their results, and no correlation was found between concentrations of particles > 500 nm and of INP (Gong et al., 2019).
Literature:
Chen et al., 2018: Chen, J., Z. Wu, S. Augustin-Bauditz, S. Grawe, M. Hartmann, X. Pei, Z. Liu, D. Ji, and H. Wex (2018), Ice nucleating particle concentrations unaffected by urban air pollution in Beijing, China, Atmos. Chem. Phys., 18, 3523–3539, doi:10.5194/acp-18-3523-2018.
Chen et al., 2021: Chen, J., Wu, Z., Zhao, X., Wang, Y., Chen, J., Qiu, Y., Zong, T., Chen, H., Wang, B., Lin, P., Liu, W., Guo, S., Yao, M., Zeng, L., Wex, H., Liu, X., Hu, M., and Li, S.-M. (2021), Atmospheric humic-like substances (HULIS) act as ice-nucleating particles, Geophys. Res. Lett., 48(e2021GL092443), doi:10.1029/2021GL092443.
Gong et al., 2019: Gong, X., H. Wex, T. Müller, A. Wiedensohler, K. Höhler, K. Kandler, N. Ma, B. Dietel, T. Schiebel, O. Möhler, and F. Stratmann (2019), Characterization of aerosol properties at Cyprus, focusing on cloud condensation nuclei and ice nucleating particles, Atmos. Chem. Phys., 19, 10883-10900, doi:10.5194/acp-19-10883-2019.
Gong et al., 2020: Gong, X., H. Wex, M. van Pinxteren, N. Triesch, K. W. Fomba, J. Lubitz, C. Stolle, B. Robinson, T. Müller, H. Herrmann, and F. Stratmann (2020), Characterization of aerosol particles at Cape Verde close to sea and cloud level heights - Part 2: ice nucleating particles in air, cloud and seawater, Atmos. Chem. Phys., 20, 1451-1468, doi:10.5194/acp-20-1451-2020.
Gong et al., 2022: Gong, X., M. Radenz, H. Wex, P. Seifert, F. Ataei, S. Henning, H. Baars, B. Barja, A. Ansmann, and F. Stratmann (2022), Significant continental source of ice-nucleating particles at the tip of Chile’s southernmost Patagonia region, Atmos. Chem. Phys., 22, 10505-10525, doi:10.5194/acp-22-10505-2022.
Hartmann et al., 2019: Hartmann, M., T. Blunier, S. O. Brügger, J. Schmale, M. Schwikowski, A. Vogel, H. Wex, and F. Stratmann (2019), Variation of ice nucleating particles in the European Arctic over the last centuries, Geophys. Res. Lett., 46, doi:10.1029/2019GL082311.
Hartmann et al., 2020: Hartmann, M., K. Adachi, O. Eppers, C. Haas, A. Herber, R. Holzinger, A. Hünerbein, E. Jäkel, C. Jentzsch, M. van Pinxteren, H. Wex, S. Willmes, and F. Stratmann (2020), Wintertime airborne measurements of ice nucleating particles in the high Arctic: a hint to a marine, biogenic source for Ice Nucleating Particles, Geophys. Res. Lett., 47(e2020GL087770), doi:10.1029/2020GL087770.
Hartmann et al., 2021: Hartmann, M., X. Gong, S. Kecorius, M. van Pinxteren, T. Vogl, A. Welti, H. Wex, S. Zeppenfeld, H. Herrmann, A. Wiedensohler, and F. Stratmann (2021), Terrestrial or marine? – Indications towards the origin of Ice Nucleating Particles during melt season in the European Arctic up to 83.7°N, Atmos. Chem. Phys., 21, 11613-11636, doi:10.5194/acp-21-11613-2021.
Knackstedt et al., 2018: Knackstedt, K. A., B. F. Moffett, S. Hartmann, H. Wex, T. C. Hill, E. D. Glasgo, L. A. Reitz, S. Augustin-Bauditz, B. F. N. Beall, G. S. Bullerjahn, J. Fröhlich-Nowoisky, S. Grawe, J. Lubitz, and R. M. L. McKay (2018), A terrestrial origin for abundant riverine nanoscale ice-nucleating particles, Environ. Sci. Technol., 12358−12367, doi:10.1021/acs.est.8b03881.
Šantl-Temkiv et al., 2019: Šantl-Temkiv, T., Lange, R., Beddows, D., Rauter, U., Pilgaard, S., Dall’Osto, M., Gunde-Cimerman, N., Massling, A., and Wex, H. (2019), Biogenic sources of Ice Nucleation Particles at the high Arctic site Villum Research Station, Environ. Sci. Technol., 53(18), 10580-10590, doi:10.1021/acs.est.9b00991.
Schmale et al., 2019: Schmale, J., A. Baccarini, I. Thurnherr, S. Henning, A. Efraim, L. Regayre, C. Bolas, M. Hartmann, A. Welti, K. Lehtipalo, F. Aemisegger, C. Tatzelt, S. Landwehr, R. L. Modini, F. Tummon, J. S. Johnson, N. Harris, M. Schnaiter, A. Toffoli, M. Derkani, N. Bukowiecki, F. Stratmann, J. Dommen, U. Baltensperger, H. Wernli, D. Rosenfeld, M. Gysel-Beer, and K. S. Carslaw (2019), Overview of the Antarctic Circumnavigation Expedition: Study of Preindustrial-like Aerosols and their Climate Effects (ACE-SPACE), BAMS, doi:10.1175/BAMS-D-18-0187.1.
Sze et al., 2022: Sze, K. C. H., H. Wex, M. Hartmann, H. Skov, A. Massling, D. Villanueva, and F. Stratmann (2022), Ice Nucleating Particles in Northern Greenland: annual cycles, biological contribution and parameterizations, Atmos. Chem. Phys. Discuss., doi:10.5194/acp-2022-761.
Tatzelt et al., 2022: Tatzelt, C., Henning, S., Welti, A., Baccarini, A., Hartmann, M., Gysel-Beer, M., van Pinxteren, M., Modini, R. L., Schmale, J., and Stratmann, F. (2021), Circum-Antarctic abundance and properties of CCN and INP, Atmos. Chem. Phys. Discuss., 21, doi:https://doi.org/10.5194/acp-2021-700.
Welti et al., 2018: Welti, A., K. Müller, Z. L. Fleming, and F. Stratmann (2018), Concentration and variability of ice nuclei in the subtropical maritime boundary layer, Atmos. Chem. Phys., 18, doi:10.5194/acp-18-5307-2018.
Welti et al., 2020: Welti, A., E. K. Bigg, P. J. DeMott, X. Gong, M. Hartmann, M. Harvey, S. Henning, P. Herenz, T. C. J. Hill, B. Hornblow, C. Leck, M. Löffler, C. S. McCluskey, A. M. Rauker, J. Schmale, C. Tatzelt, M. van Pinxteren, and F. Stratmann (2020), Ship-based measurements of ice nuclei concentrations over the Arctic, Atlantic, Pacific and Southern Ocean, Atmos. Chem. Phys., 20, doi:10.5194/acp-2020-466.
Wendisch et al., 2019: Wendisch et al. (2019), The Arctic cloud puzzle: Using ACLOUD/PASCAL multi-platform observations to unravel the role of clouds and aerosol particles in Arctic Amplification, BAMS, doi:10.1175/BAMS-D-18-0072.1
Wendisch et al., 2022: Wendisch, M. et al. (2022), Atmospheric and surface processes, and feedback mechanisms determining Arctic Amplification: A review of first results and prospects of the (AC)³ project, Bull. Amer. Meteor. Soc., doi:10.1175/BAMS-D-21-0218.1.
Wex et al., 2019: Wex, H., L. Huang, W. Zhang, H. Hung, R. Traversi, S. Becagli, R. J. Sheesley, C. E. Moffett, T. E. Barrett, R. Bossi, H. Skov, A. Hünerbein, J. Lubitz, M. Löffler, O. Linke, M. Hartmann, P. Herenz, and F. Stratmann (2019), Annual variability of ice nucleating particle concentrations at different Arctic locations, Atmos. Chem. Phys., 19, 5293–5311, doi:10.5194/acp-19-5293-2019.